Recent Advances in Quantum Dot Lasers for Silicon Photonics
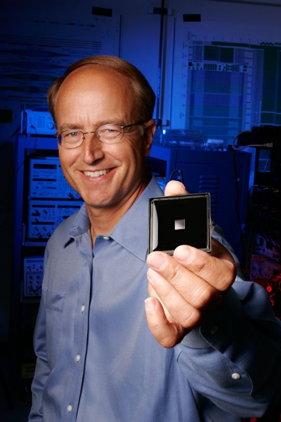
Silicon photonics is revolutionizing optical technologies by enabling scalable manufacturing of densely integrated photonic systems on a chip. Such systems will be utilized in datacenter and high-performance computing interconnects, chemical and biomolecular sensors, and in numerous other applications where small form factor, low power consumption, and multi-functionality are desirable. Perhaps most desirable amongst these capabilities, is the energy efficiency that can be obtained through displacing electrical interconnects within datacenters. Datacenters currently consume >2.5% of the electricity produced in the United States, and the vast majority of that consumption could be eliminated with optical interconnects. The only drawback to silicon photonics is the inability of silicon to form an efficient light emitter, and silicon’s inherent crystalline incompatibility with III-V materials, which make excellent light sources.
The incompatibility of III-V’s with Si has been historically overcome by fabricating the laser material stack separately on a native III-V substrate which is then bonded to a silicon-on-insulator substrate already patterned with other photonic components, such as waveguides, modulators, and detectors. This technique was pioneered within the Bowers group in 2007 and has been commercialized by multiple companies including Intel, Hewlett-Packard Enterprise, and Juniper Networks. The primary limitation of this technology is that the III-V substrates required for making the laser are expensive and the maximum wafer size is less than half that of what is available on Si thus limiting manufacturing scalability.
Recently we have made several considerable advances in the growth and fabrication of III-V lasers directly on a silicon substrate through the use of quantum dot active layers for light emission that show promise for commercial viability. Quantum dots are nanoscale inclusions of one semiconductor material within a matrix composed of a second material. If the materials are chosen such that the bandgap of the matrix material is larger than that of the inclusion, then the size of the inclusions will lead to useful quantum mechanical properties similar to the textbook particle-in-a-box scenario. These properties make for extremely efficient light emitters that are insensitive to crystalline defects, have improved performance at high temperatures, and exhibit unique dynamic properties beneficial to photonic integrated circuits.
Key to our recent advances are the development of low defect density III-V materials on Si and careful optimization of the quantum dot active layer growth. Through the combined efforts of former student, Alan Liu, current students, Justin Norman and Chen Shang, and postdoc, Daehwan Jung, more than 500 samples have been grown to optimize our material. The results have led to III-V layers grown on Si with near record low defect density of 6×106 cm-2 and world record laser performance around the commonly used datacom wavelength of 1300 nm. Our most recent results, with the help of new postdoc, Yating Wan, include the demonstration of micron-scale ring lasers with record low threshold currents under 1 mA and Fabry-Perot ridge lasers with threshold currents as low as 4.8 mA, wall-plug efficiency as high as 38%, and single-facet output powers up to 185 mW with devices operating continuous wave above 100°C. Through collaborations with NAsPIII/V, GmbH, Hong Kong University of Science and Technology, and AIM Photonics we have achieved all of the previously mentioned results using industry standard on-axis silicon substrates.
The performance of our lasers goes beyond their static properties with extrapolated device lifetimes--under continuous operation at 35°C and twice the threshold current density--of >100,000,000 hours having been demonstrated. The previous world record at these conditions was ~4,000 hours. In order to prove the viability of our devices in a datacenter or high performance computing environment, lifetime testing is currently underway at an elevated temperature of 65°C.
While we are still actively improving our laser materials, our efforts have grown to encompass more complex implementations focused on high speed modulation, mode-locking, waveguide integration, and the utilization of quantum dots in other components including amplifiers, modulators, and detectors. Each of these components will form the building blocks of future, all-epitaxial, quantum dot-based photonic integrated circuits on silicon.
Justin C. Norman, John E. Bowers