Kaustav Banerjee yields a smaller, higher-performing alternative to the classic inductor
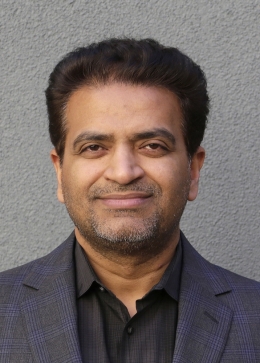
A basic building block of modern technology, inductors are everywhere: cellphones, laptops, radios, televisions, cars. And surprisingly, they are essentially the same today as in 1831, when they were first created by English scientist Michael Faraday.
The particularly large size of inductors made according to Faraday’s design are a limiting factor in delivering the miniaturized devices that will help realize the potential of the Internet of Things, which promises to connect people to some 50 billion objects by 2020. That lofty goal is expected to have an estimated economic impact between $2.7 and $6.2 trillion annually by 2025.
Now, a team at UC Santa Barbara, led by Kaustav Banerjee, a professor in the Department of Electrical and Computer Engineering, has taken a materials-based approach to reinventing this fundamental component of modern electronics. The findings appear in the journal Nature Electronics.
Artist's depiction of the intercalated multilayer-graphene inductor (center blue spiral). Background images show its predecessors.
Photo Credit: PETER ALLEN
Banerjee and his UCSB team — lead author Jiahao Kang, Junkai Jiang, Xuejun Xie, Jae Hwan Chu and Wei Liu, all members of his Nanoelectronics Research Lab — worked with colleagues from Shibaura Institute of Technology in Japan and Shanghai Jiao Tong University in China to exploit the phenomenon of kinetic inductance to demonstrate a fundamentally different kind of inductor.
All inductors generate both magnetic and kinetic inductance, but in typical metal conductors, the kinetic inductance is so small as to be unnoticeable. “The theory of kinetic inductance has long been known in condensed-matter physics, but nobody ever used it for inductors, because in conventional metallic conductors, kinetic inductance is negligible,” Banerjee explained.
Unlike magnetic inductance, kinetic inductance does not depend on the surface area of the inductor. Rather, kinetic inductance resists current fluctuations that alter the velocity of the electrons, and the electrons resist such change according to Newton’s law of inertia.
Historically, as the technology of transistors and interconnects that link them has advanced, the elements have become smaller. But the inductor, which in its simplest form is a metallic coil wound around a core material, has been the exception.
“On-chip inductors based on magnetic inductance cannot be made smaller in the same way transistors or interconnects scale, because you need a certain amount of surface area to get a certain magnetic flux or inductance value,” explained lead author Kang, who recently completed his Ph.D. under Banerjee’s supervision.
The UCSB team designed a new kind of spiral inductor comprised of multiple layers of graphene. Single-layer graphene exhibits a linear electronic band structure and a correspondingly large momentum relaxation time (MRT) — a few picoseconds or higher compared to that of conventional metallic conductors (like copper used in traditional on-chip inductors), which ranges from 1/1000 to 1/100 of a picosecond. But single-layer graphene has too much resistance for application on an inductor.
However, multilayer graphene offers a partial solution by providing lower resistance, but interlayer couplings cause its MRT to be insufficiently small. The researchers overcame that dilemma with a unique solution: They chemically inserted bromine atoms between the graphene layers — a process called intercalation — that not only further reduced resistance but also separated the graphene layers just enough to essentially decouple them, extending the MRT and thereby increasing kinetic inductance.
The revolutionary inductor, which works in the 10-50 GHz range, offers one-and-a-half times the inductance density of a traditional inductor, leading to a one-third reduction in area while also providing extremely high efficiency. Previously, high inductance and reduced size had been an elusive combination.
“There is plenty of room to increase the inductance density further by increasing the efficiency of the intercalation process, which we are now exploring,” said co-author Jiang.
“We essentially engineered a new nanomaterial to bring forward the previously ‘hidden physics’ of kinetic inductance at room temperature and in a range of operating frequencies targeted for next-generation wireless communications,” Banerjee added.
While Faraday’s version of the inductor proved crucial for practical large scale use of electricity for power and telegraph communication, Banerjee’s version could pave the way for next-generation communications, sensing and energy storage for smarter living.