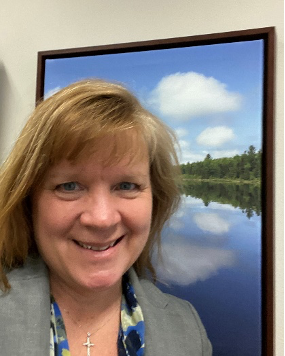
Abstract
Photonic isolators are essentially diodes for optical signals, and as such they prevent backward propagation of light as needed, most frequently in front of lasers as protection from reflected power. After introducing the phenomenon of non-reciprocity, the operation of commercial (bulk) isolators will be discussed, including magneto-optical Faraday rotation which is prevalent in iron garnets. Although commercial isolators have their own markets, future hyperscale data needs are moving ever closer to integrated photonics as pluggable transceivers are replaced by co-packaged optics. In these markets, the laser is the most sensitive element, and passive (zero power) isolators have been demonstrated using magneto-optical garnet films [1] in one of two configurations. The first configuration involves the use of permanent magnets to produce a transverse magnetization in the garnet, which in turn causes a non-reciprocal phase shift (NRPS) in the propagating signal. Isolation is then achieved by either a Mach Zhender Interferometer [2] or a Ring Resonator [3]. However, these devices only work in TM-mode operation unless bulky polarization converters [4] or difficult sidewall coating are employed [5]. The second configuration involves longitudinal magnetization for non-reciprocal mode conversion (NRMC) [6]. This magnetization is the remnant state of the garnet, so no additional magnet is needed. In addition, cerium-doped terbium iron garnets (Ce:TbIG) with high Faraday rotation but low saturation magnetization can be designed to reduce dipole fields for zero device cross talk. In NRMC devices, quasi-phase matching can be used to offset any waveguide bi-refringence. The resulting devices are one-dimensional and polarization diverse (TM- and TE-mode operation), which benefits all current on-chip lasers (TE mode) [7,8]. The only downside to integrated garnets is their high crystallization temperatures. Monolithic integration is possible if the garnet is incorporated before temperature sensitive materials. Alternatively, large scale transfer lithography has been recently demonstrated where a simple anneal induced strain-mediated diffusion of vacancies to create an exfoliation gap between Ce:TbIG films and their growth wafers [9]. Commercial grade devices are currently being produced and tested for fully integrated Si-photonic isolators.
- Sung, S. Y., Qi, X., & Stadler, B. J. (2005). Integrating yttrium iron garnet onto nongarnet substrates with faster deposition rates and high reliability. Applied Physics Letters, 87, 121111.
- Stadler, B. J., & Mizumoto, T. (2013). Integrated magneto-optical materials and isolators: a review. IEEE Photonics Journal, 6(1), 1-15.
- Bi, L., Hu, J., Jiang, P., Kim, D. H., Dionne, G. F., Kimerling, L. C., & Ross, C. A. (2011). On-chip optical isolation in monolithically integrated non-reciprocal optical resonators. Nature Photonics, 5(12), 758-762.
- Ghosh, S., Keyvaninia, S., Shirato, Y., Mizumoto, T., Roelkens, G., & Baets, R. (2013). Optical isolator for TE polarized light realized by adhesive bonding of Ce: YIG on silicon-on-insulator waveguide circuits. IEEE Photonics Journal, 5(3), 6601108-6601108.
- Zhang, Y., Du, Q., Wang, C., Fakhrul, T., Liu, S., Deng, L., Huang, D., Pintus, P., Bowers, J., Ross, C.A. and Hu, J. (2019). Monolithic integration of broadband optical isolators for polarization-diverse silicon photonics. Optica, 6(4), pp.473-478.
- Dulal, P., Block, A.D., Gage, T.E., Haldren, H.A., Sung, S.Y., Hutchings, D.C. and Stadler, B.J., (2016). Optimized magneto-optical isolator designs inspired by seedlayer-free terbium iron garnets with opposite chirality. ACS Photonics, 3(10), pp.1818-1825.
- Zhang C, Dulal P, Stadler BJ, Hutchings DC. Monolithically-integrated TE-mode 1D silicon-on-insulator isolators using seedlayer-free garnet. Scientific reports. (2017) Jul 19;7(1):1-8.
- Srinivasan K, Zhang C, Dulal P, Radu C, Gage TE, Hutchings DC, Stadler BJ. High-gyrotropy seedlayer-free Ce: TbIG for monolithic laser-matched SOI optical isolators. ACS Photonics. 2019 Sep 6;6(10):2455-61.
- Srinivasan, Schwarz, Myers, Seaton, Stadler (2021). Diffusion-Driven Exfoliation of Magneto-Optical Garnet Nanosheets: Implications for Low Thermal Budget Integration in Si Photonics, ACS Applied Nano Materials (2021). 10.1021/acsanm.1c02459
Biography
Bethanie Stadler is a Professor and Associate Head of Electrical & Computer Engineering at the University of Minnesota, where she also holds the CS&E Distinguished Professorship and is on the Graduate Faculty of both Chemical Engineering & Materials Science and Mechanical Engineering. She earned her PhD from MIT and a BS from Case Western Reserve University. She is a Fellow of the Materials Research Society (MRS). Stadler works on magnetic nanowires for applications in RF design and biomedicine, including contact-free barcoded bandaids for deep labeling that is detected using novel magnetic readout. She also works on magneto-optical garnets for integrated photonics, including one-dimensional magnet-free isolators. Stadler has been a visiting professor at IMEC and KU Leuven in Belgium and also at Wright Patterson Air Force Base in Dayton Ohio. In 2015, Stadler was an IEEE Magnetics Society Distinguished Lecturer. She has taught at the IEEE Magnetic Summer School in India and Italy, and hosted the school in Minnesota in 2015. In serving MRS, Stadler has been a meeting chair (Fall 2004), director on the board, secretary and chair of the program development subcommittee. She has also served as chair the program committee and in many other roles for the IEEE Magnetics Society, and she will be the General Co-Chair of Intermag 2023 in Sendai, Japan.