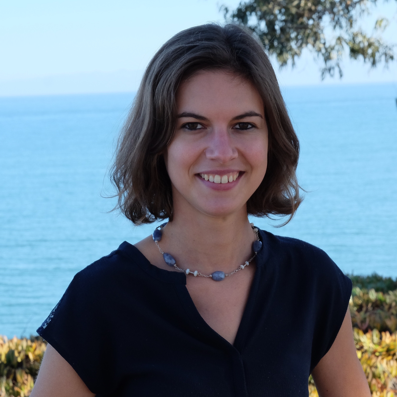
Abstract
Designing highly performant electrochemical devices, such as rechargeable batteries, requires an in-depth understanding of the electronic and structural processes at play during normal operation and potentially leading to performance degradation.
While X-ray scattering and X-ray absorption techniques can provide information on long-range structural changes and oxidation states averaged over the whole structure, the combination of solid-state Nuclear Magnetic Resonance spectroscopy (ssNMR) and first principles calculations enables to monitor local structural and redox processes. These local probes are particularly important for the investigation of (paramagnetic) electrode materials which are either intrinsically disordered or disorder during electrochemical cycling.
Sodium(Na)-ion batteries have emerged as cheaper, more sustainable alternatives to lithium(Li)-ion batteries. The first part of this talk will focus on sodium transition metal oxide cathodes. These layered compounds have demonstrated volumetric energy densities comparable to that of current commercial lithium-ion cathodes, yet, phase transitions upon electrochemical cycling limit their cycle life and are a major obstacle to their commercialization. ssNMR and theory prove to be powerful diagnostic tools, providing invaluable insight into the migration of species and the kinetics of Na+ ionic motion within the crystal lattice as a function of (dis)charge, into the effect of transition metal substitution on structural stability and electrochemical performance, and into the nature and amount of structural defects in the material.
Cation-disordered lithium transition metal oxide cathodes have emerged as a new class of high energy density Li-ion cathodes. Unlike their commercialized layered counterparts based on Co, Ni or Mn, these disordered compounds can be stabilized using Earth-abundant transition metal species, such as Fe and Ti. In the second part of this talk, we will see how the study of such complex materials necessitates the combined use of advanced theoretical (first principles and Monte Carlo simulations) and experimental (ssNMR) tools to elucidate the impact of partial fluorine substitution for oxygen on the local redox chemistry and on short-range order.
Biography
Raphaële Clément received her B.A. and M.Sc. in Natural Sciences in 2012, as well as her Ph.D. in Chemistry in 2016, from the University of Cambridge. Her Ph.D., supervised by Prof. Clare Grey, focused on the study of paramagnetic cathode materials for sodium-ion battery applications using solid-state Nuclear Magnetic Resonance (NMR) spectroscopy. She then joined the group of Prof. Gerbrand Ceder at UC Berkeley as a postdoc, where she developed both experimental and computational skills applicable to a range of electrochemical materials. She joined the faculty of the Materials Department at UCSB in July 2018. Her current research interests are the study of structural and redox processes in electrochemical materials using magnetic resonance techniques and theoretical tools. She is particularly interested in the development of Electron Paramagnetic Resonance (EPR) and NMR tools for the study of functional materials operando or in situ, e.g. to capture transient, metastable intermediates forming during normal operation, to study the kinetics of processes, and for full device analysis.