Professor Chris Van de Walle’s group elucidates recombination mechanisms in hybrid perovskites
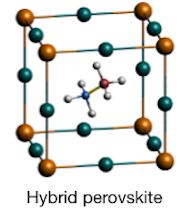
Hybrid perovskites are a group of materials that combine organic molecules with an inorganic framework in a perovskite lattice structure. Even though solar cells based on these materials were demonstrated less than 10 years ago, solar conversion efficiencies have already shot up to values exceeding 22%. Interestingly, the fundamental mechanisms that are responsible for the high efficiency are not yet properly understood and are vigorously debated. A thorough understanding of these mechanisms is essential to enable further improvements. The Van de Walle group recently reported breakthroughs in two papers published in high-impact journals. [X. Zhang, J.-X. Shen, W. Wang, and C. G. Van de Walle, ACS Energy Lett. 3, 2329 (2018) and J.-X. Shen, X. Zhang, S. Das, E. Kioupakis, and C. G. Van de Walle, Adv. Energy Mater. 8, 1801027 (2018)]
A number of research groups previously attributed the high efficiency of the hybrid perovskites to an indirect band gap originating from strong spin-orbit coupling. It was argued that the indirect nature of the gap suppresses radiative recombination between electrons and holes, and thus minimizes undesirable carrier recombination. Postdoc Xie Zhang and PhD student Jimmy-Xuan Shen (who has since graduated) demonstrated that this was incorrect. They developed a cutting-edge first-principles approach to accurately determine the spin texture of the band edges and quantitatively compute the radiative recombination rates. For methylammonium lead iodide (the prototype hybrid perovskite commonly referred to as MAPI) they found that the radiative recombination is actually as strong as in conventional direct-gap semiconductors. “This result should put an end to misguided attempts to analyze and design device characteristics based on erroneous assumptions about the recombination rate,” said Zhang.
Strong radiative recombination means that these materials are also useful for light-emitting diode (LED) applications. However, current densities in LEDs are much higher than in solar cells, and at high carrier concentrations nonradiative recombination processes can become detrimental. Such nonradiative losses have been observed, but experimentally it is not possible to identify the microscopic origins. Shen and Zhang built on the unique expertise in the Van de Walle to accurately compute the recombination rate from first principles. They also managed to precisely link the rate to features in the electronic structure. “Auger recombination is a process in which two carriers recombine across the band gap and the excess energy is transferred to a third carrier,” explained Shen. “We found that the Auger coefficient in MAPI is unexpectedly large: two orders of magnitude larger than in other semiconductors with comparable band gaps!” The researchers identified two distinct features of the material that are responsible: first, a resonance between the band gap and the spin-orbit-induced splitting of the conduction bands, and second, the presence of structural distortions that promote the Auger process. “Our work demonstrates that Auger losses can be suppressed if lattice distortions are reduced, and we propose specific approaches for achieving this in real materials,” commented Van de Walle.