Professor Van de Walle's group gains new insights in doping of transparent conductors
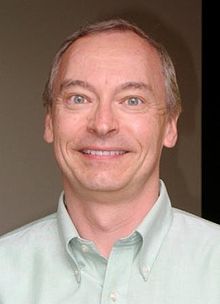
Materials that are simultaneously transparent and electrically conductive play a key role in light-emitting diodes, solar cells, and displays. For optoelectronic devices to be able to emit or absorb light,it is important that the electrical contacts at the top of the device are optically transparent. While metals are the best electrical conductors available, they are also opaque. Most optically transparent materials, on the other hand, are insulating and cannot be used as electrodes. Conducting oxides strike the best balance between optical transparency and electrical conductivity. Their wide band gaps prevent absorption of visible light by excitation of electrons across the gap. At the same time, dopant atoms provide additional electrons in the conduction band that enable electrical conductivity.
The Van de Walle group has been studying both aspects of the problem: transparency and conductivity. The electrons in the conduction band can absorb light by being excited to higher conduction-band states, and this puts a fundamental limit on the transparency of the material; these limits were examined in previous work [e.g., H. Peelaers, E. Kioupakis, and C.G. Van de Walle, Phys. Rev. B 92, 235201 (2015) and H. Peelaers and C. G. Van de Walle, Appl. Phys. Lett. 111, 182104 (2017)].
Control of conductivity in transparent conductors depends on identifying suitable dopants, and examining potential sources of compensation. In recent work, Van de Walle and coworkers have turned their attention to barium stannate (BaSnO3), an oxide that has come to the forefront as a promising new transparent conductor. However, its conductivity has been difficult to control. The problem is even more severe in SrSnO3, a closely related material that has an even wider band gap; this feature could make it useful as a transparent conductor for UV optoelectronics, but also in modulation-doped heterostructures that could enable devices based on a two-dimensional electron gas in BaSnO3.
Postdoc Leigh Weston and coworkers performed comprehensive calculations of point defects and impurities, using cutting-edge first-principles techniques, with the goal of determining the mechanisms that limit doping. They found that atomic-scale defects, such as vacancies in the crystal lattice, are responsible, but their formation can be controlled by tailoring the growth conditions. A comparison of the results for BaSnO3, SrSnO3, and CaSnO3 provided insights into the trends across a series of materials with different band gaps. Calcium stannate was found to always be insulating, but guidelines were provided for maximizing the conductivity of the strontium compound.
The figure below shows the alignment of the band structures of barium stannate, strontium stannate, and calcium stannate, indicating their potential for n-type doping using lanthanum donors. The position of the Fermi level (colored bars) is indicated under oxygen-rich and oxygen-poor conditions. A higher Fermi-level position indicates highern-type doping; a Fermi-level position below the conduction band indicates significant charge compensation.
The work was published in Physical Review B and highlighted as an Editors’ Suggestion[”Origins of n-type doping difficulties in perovskite stannates”, L. Weston, L. Bjaalie, K. Krishnaswamy, and C. G. Van de Walle, Phys. Rev. B 97, 054112 (2018)].